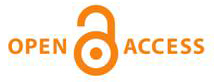
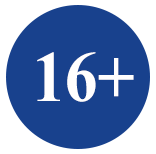
Legal and postal addresses of the founder and publisher: Northern (Arctic) Federal University named after M.V. Lomonosov, Naberezhnaya Severnoy Dviny, 17, Arkhangelsk, 163002, Russian Federation
Editorial office address: Journal of Medical and Biological Research, 56 ul. Uritskogo, Arkhangelsk
Phone: (818-2) 21-61-00, ext.18-20
E-mail: vestnik_med@narfu.ru
https://vestnikmed.ru/en/
|
The Role of the Acetylcholine System and Its Components in the Development of Post-COVID Syndromes (Review). 240-252
|
 |
Section: Review articles
Download
(pdf, 0.3MB )
UDC
[612.8.04+612.81]:[616-008.64+616-009]
DOI
10.37482/2687-1491-Z182
Abstract
According to the latest data, COVID-19 is classified as a respiratory virus. However, it has been proven to cause a significant multi-organ dysfunction. Despite the increase in the effectiveness of treatment tactics, a post-COVID-19 symptom complex has been observed in patients after recovery, manifesting itself as cephalalgia, brain fog, high fever, muscle weakness and a decrease (or increase) in arterial pressure. To describe this condition, a clinical characteristic has been proposed: post-acute COVID-19 syndrome (PACS). Approximately 57 % of patients hospitalized with COVID-19 have symptoms of PACS even one year after initial infection with COVID-19. This pathological condition is being actively studied, but the question of the causes of PACS and its development mechanisms remains open. One of the possible reasons behind this symptomatology, in our opinion, is a disorder of the body’s acetylcholine system and its components. The acetylcholine system plays an integral role in various physiological and pathophysiological processes, such as the regulation of the muscular system, immune and inflammatory reactions, wound healing, as well as the development of cardiovascular, respiratory and other diseases. A key way of translating acetylcholine signals in the body is through the use of neurotransmission via a chemical synapse. Based on current literature data, there is reason to believe that viral invasion can significantly change the functional activity of the vagus nerve by disrupting signal transmission in a synapse. We believe that the hyperimmune response caused by COVID-19 triggers a chain of pathological mechanisms that are associated with disrupted nitric oxide production and imbalance in acetylcholine and its receptors. An understanding of these processes may open up prospects for improving the effectiveness of treatment and rehabilitation of patients with COVID-19.
Keywords
acetylcholine system disorder, neuromuscular junction, synaptic signalling disorder, consequences of COVID-19, post-COVID syndrome
References
- WHO COVID-19 Dashboard. Available at: https://covid19.who.int/ (accessed: 4 April 2023).
- Huang C., Wang Y., Li X., Ren L., Zhao J., Hu Y., Zhang L., Fan G., Xu J., Gu X., Cheng Z., Yu T., Xia J., Wei Y., Wu W., Xie X., Yin W., Li X., Liu M., Xiao Y., Gao H., Guo L., Xie J., Wang G., Jiang R., Gao H., Jin Q., Wang J., Cao B. Clinical Features of Patients Infected with 2019 Novel Coronavirus in Wuhan, China. Lancet, 2020, vol. 395, no. 10223, pp. 497–506. https://doi.org/10.1016/s0140-6736(20)30183-5
- Yahia A.I.O. Liver Injury and Dysfunction Associated with COVID-19: A Review Article. Clin. Lab., 2022, vol. 68, no. 1. https://doi.org/10.7754/clin.lab.2021.210535
- Ayoubkhani D., Khunti K., Nafilyan V., Maddox T., Humberstone B., Diamond I., Banerjee A. Post-Covid Syndrome in Individuals Admitted to Hospital with Covid-19: Retrospective Cohort Study. BMJ, 2021, vol. 372. Art. no. n693. https://doi.org/10.1136/bmj.n693
- Desai A.D., Lavelle M., Boursiquot B.C., Wan E.Y. Long-Term Complications of COVID-19. Am. J. Physiol. Cell Physiol., 2022, vol. 322, no. 1, pp. C1–C11. https://doi.org/10.1152/ajpcell.00375.2021
- Tobler D.L., Pruzansky A.J., Naderi S., Ambrosy A.P., Slade J.J. Long-Term Cardiovascular Effects of COVID-19: Emerging Data Relevant to the Cardiovascular Clinician. Curr. Atheroscler. Rep., 2022, vol. 24, no. 7, pp. 563–570. https://doi.org/10.1007/s11883-022-01032-8
- Willi S., Lüthold R., Hunt A., Hänggi N.V., Sejdiu D., Scaff C., Bender N., Staub K., Schlagenhauf P. COVID-19 Sequelae in Adults Aged Less Than 50 Years: A Systematic Review. Travel Med. Infect. Dis., 2021, vol. 40. Art. no. 101995. https://doi.org/10.1016/j.tmaid.2021.101995
- Haam J., Yakel J.L. Cholinergic Modulation of the Hippocampal Region and Memory Function. J. Neurochem., 2017, vol. 142, suppl. 2, pp. 111–121. https://doi.org/10.1111/jnc.14052
- Mesulam M. The Cholinergic Lesion of Alzheimer’s Disease: Pivotal Factor or Side Show? Learn. Mem., 2004, vol. 11, no. 1, pp. 43–49. https://doi.org/10.1101/lm.69204
- Picciotto M.R., Higley M.J., Mineur Y.S. Acetylcholine as а Neuromodulator: Cholinergic Signaling Shapes Nervous System Function and Behavior. Neuron, 2012, vol. 76, no. 1, pp. 116–129. https://doi.org/10.1016/j.neuron.2012.08.036
- Ahmed N.Y., Knowles R., Dehorter N. New Insights into Cholinergic Neuron Diversity. Front. Mol. Neurosci., 2019, no. 12. Art. no. 204. https://doi.org/10.3389/fnmol.2019.00204
- Rima M., Lattouf Y., Younes M.A., Bullier E., Legendre P., Mangin J.-M., Hong E. Dynamic Regulation of the Cholinergic System in the Spinal Central Nervous System. Sci. Rep., 2020, vol. 10. Art. no. 15338. https://doi.org/10.1038/s41598-020-72524-3
- Bosmans G., Shimizu Bassi G., Florens M., Gonzalez-Dominguez E., Matteoli G., Boeckxstaens G.E. Cholinergic Modulation of Type 2 Immune Responses. Front. Immunol., 2017, vol. 8. Art. no. 1873. https://doi.org/10.3389/fimmu.2017.01873
- Scott G.D., Fryer A.D. Role of Parasympathetic Nerves and Muscarinic Receptors in Allergy and Asthma. Chem. Immunol. Allergy, 2012, no. 98, pp. 48–69. https://doi.org/10.1159/000336498
- Saw E.L., Pearson J.T., Schwenke D.O., Munasinghe P.E., Tsuchimochi H., Rawal S., Coffey S., Davis P., Bunton R., Van Hout I., Kai Y., Williams M.J.A., Kakinuma Y., Fronius M., Katare R. Activation of the Cardiac Non-Neuronal Cholinergic System Prevents the Development of Diabetes-Associated Cardiovascular Complications. Cardiovasc. Diabetol., 2021, vol. 20, no. 1. Art. no. 50. https://doi.org/10.1186/s12933-021-01231-8
- Eccles J.C., Fatt P., Koketsu K. Cholinergic and Inhibitory Synapses in a Pathway from Motor-Axon Collaterals to Motoneurones. J. Physiol., 1954, vol. 126, no. 3, pp. 524–562. https://doi.org/10.1113/jphysiol.1954.sp005226
- Chen J., Mizushige T., Nishimune H. Active Zone Density Is Conserved During Synaptic Growth but Impaired in Aged Mice. J. Comp. Neurol., 2012, vol. 520, no. 2, pp. 434–452. https://doi.org/10.1002/cne.22764
- Schiavo G., Stenbeck G., Rothman J.E., Söllner T.H. Binding of the Synaptic Vesicle v-SNARE, Synaptotagmin, to the Plasma Membrane t-SNARE, SNAP-25, Can Explain Docked Vesicles at Neurotoxin-Treated Synapses. Proc. Natl. Acad. Sci. USA, 1997, vol. 94, no. 3, pp. 997–1001. https://doi.org/10.1073/pnas.94.3.997
- White D.N., Stowell M.H.B. Room for Two: The Synaptophysin/Synaptobrevin Complex. Front. Synaptic Neurosci., 2021, vol. 13. Art. no. 740318. https://doi.org/10.3389/fnsyn.2021.740318
- Krause M., Wernig A. The Distribution of Acetylcholine Receptors in the Normal and Denervated Neuromuscular Junction of the Frog. J. Neurocytol., 1985, vol. 14, no. 5, pp. 765–780. https://doi.org/10.1007/BF01170827
- Fujita A., Cheng J., Tauchi-Sato K., Takenawa T., Fujimoto T. A Distinct Pool of Phosphatidylinositol 4,5-Bisphosphate in Caveolae Revealed by a Nanoscale Labeling Technique. Proc. Natl. Acad. Sci. USA, 2009, vol. 106, no. 23, pp. 9256–9261. https://doi.org/10.1073/pnas.0900216106
- Petrov A.M., Zefirov A.L. Kholesterin i lipidnye plotiki biologicheskikh membran. Rol’ v sekretsii, retseptsii i funktsionirovanii ionnykh kanalov [Cholesterol and Lipid Rafts in the Biological Membranes. Role in the Release, Reception and Ion Channel Functions]. Uspekhi fiziologicheskikh nauk, 2013, vol. 44, no. 1, pp. 17–38.
- Gazzerro E., Sotgia F., Bruno C., Lisanti M.P., Minetti C. Caveolinopathies: From the Biology of Caveolin-3 to Human Diseases. Eur. J. Hum. Genet., 2010, vol. 18, no. 2, pp. 137–145. https://doi.org/10.1038/ejhg.2009.103
- Hausser A., Schlett K. Coordination of AMPA Receptor Trafficking by Rab GTPases. Small GTPases, 2019, vol. 10, no. 6, pp. 419–432. https://doi.org/10.1080/21541248.2017.1337546
- Zakany F., Kovacs T., Panyi G., Varga Z. Direct and Indirect Cholesterol Effects on Membrane Proteins with Special Focus on Potassium Channels. Biochim. Biophys. Acta Mol. Cell Biol. Lipids, 2020, vol. 1865, no. 8. Art. no. 158706. https://doi.org/10.1016/j.bbalip.2020.158706
- Kravtsova V.V., Matchkov V.V., Bouzinova E.V., Vasiliev A.N., Razgovorova I.A., Heiny J.A., Krivoi I.I. Isoform-Specific Na,K-ATPase Alterations Precede Disuse-Induced Atrophy of Rat Soleus Muscle. Biomed. Res. Int., 2015, vol. 2015. Art. no. 720172. https://doi.org/10.1155/2015/720172
- McHardy S.F., Wang H.L., McCowen S.V., Valdez M.C. Recent Advances in Acetylcholinesterase Inhibitors and Reactivators: An Update on the Patent Literature (2012–2015). Expert Opin. Ther. Pat., 2017, vol. 27, no. 4, pp. 455–476. https://doi.org/10.1080/13543776.2017.1272571
- Bonaz B., Sinniger V., Pellissier S. Therapeutic Potential of Vagus Nerve Stimulation for Inflammatory Bowel Diseases. Front. Neurosci., 2021, vol. 15. Art. no. 650971. https://doi.org/10.3389/fnins.2021.650971
- de Jonge W.J., Ulloa L. The Alpha7 Nicotinic Acetylcholine Receptor as a Pharmacological Target for Inflammation. Br. J. Pharmacol., 2007, vol. 151, no. 7, pp. 915–929. https://doi.org/10.1038/sj.bjp.0707264
- Brenner H.R., Akaaboune M. Recycling of Acetylcholine Receptors at Ectopic Postsynaptic Clusters Induced by Exogenous Agrin in Living Rats. Dev. Biol., 2014, vol. 394, no. 1, pp. 122–128. https://doi.org/10.1016/j.ydbio.2014.07.018
- Barrantes F.J. Cell-Surface Translational Dynamics of Nicotinic Acetylcholine Receptors. Front. Synaptic Neurosci., 2014, vol. 6. Art. no. 25. https://doi.org/10.3389/fnsyn.2014.00025
- Karimi N., Okhovat A.A., Ziaadini B., Haghi Ashtiani B., Nafissi S., Fatehi F. Myasthenia Gravis Associated with Novel Coronavirus 2019 Infection: A Report of Three Cases. Clin. Neurol. Neurosurg., 2021, vol. 208. Art. no. 106834. https://doi.org/10.1016/j.clineuro.2021.106834
- Alexandris N., Lagoumintzis G., Chasapis C.T., Leonidas D.D., Papadopoulos G.E., Tzartos S.J., Tsatsakis A., Eliopoulos E., Poulas K., Farsalinos K. Nicotinic Cholinergic System and COVID-19: In silico Evaluation of Nicotinic Acetylcholine Receptor Agonists as Potential Therapeutic Interventions. Toxicol. Rep., 2020, vol. 8, pp. 73–83. https://doi.org/10.1016/j.toxrep.2020.12.013
- Bruneau E.G., Akaaboune M. The Dynamics of Recycled Acetylcholine Receptors at the Neuromuscular Junction in vivo. Development, 2006, vol. 133, no. 22, pp. 4485–4493. https://doi.org/10.1242/dev.02619
- Lück G., Hoch W., Hopf C., Blottner D. Nitric Oxide Synthase (NOS-1) Coclustered with Agrin-Induced AChR-Specializations on Cultured Skeletal Myotubes. Mol. Cell. Neurosci., 2000, vol. 16, no. 3, pp. 269–281. https://doi.org/10.1006/mcne.2000.0873
- Klyachko V.A., Ahern G.P., Jackson M.B. cGMP-Mediated Facilitation in Nerve Terminals by Enhancement of the Spike Afterhyperpolarization. Neuron, 2001, vol. 31, no. 6, pp. 1015–1025. https://doi.org/10.1016/s0896-6273(01)00449-4
- Sayed N., Baskaran P., Ma X., van den Akker F., Beuve A. Desensitization of Soluble Guanylyl Cyclase, the NO Receptor, by S-Nitrosylation. Proc. Natl. Acad. Sci. USA, 2007, vol. 104, no. 30, pp. 12312–12317. https://doi.org/10.1073/pnas.0703944104
- Proskurina S.E. Vliyanie oksida azota (NO) na aktivnost’ fermenta atsetilkholinesterazy v nervno-myshechnom sinapse krysy [Effect of Nitric Oxide (NO) on the Activity of the Acetylcholinesterase Enzyme in Rat Neuromuscular Junction: Diss.]. Kazan, 2016. 134 p.
- Petrov K.A., Malomouzh A.I., Kovyazina I.V., Krejci E., Nikitashina A.D., Proskurina S.E., Zobov V.V., Nikolsky E.E. Regulation of Acetylcholinesterase Activity by Nitric Oxide in Rat Neuromuscular Junction via N-Methyl- D-Aspartate Receptor Activation. Eur. J. Neurosci., 2013, vol. 37, no. 2, pp. 181–189. https://doi.org/10.1111/ejn.12029
- Rosas-Ballina M., Ochani M., Parrish W.R., Ochani K., Harris Y.T., Huston J.M., Chavan S., Tracey K.J. Splenic Nerve Is Required for Cholinergic Antiinflammatory Pathway Control of TNF in Endotoxemia. Proc. Natl. Acad. Sci. USA, 2008, vol. 105, no. 31, pp. 11008–11013. https://doi.org/10.1073/pnas.0803237105
- Balez R., Ooi L. Getting to NO Alzheimer’s Disease: Neuroprotection versus Neurotoxicity Mediated by Nitric Oxide. Oxid. Med. Cell. Longev., 2016, vol. 2016. Art. no. 3806157. https://doi.org/10.1155/2016/3806157
- Gilhus N.E., Tzartos S., Evoli A., Palace J., Burns T.M., Verschuuren J.J.G.M. Myasthenia Gravis. Nat. Rev. Dis. Primers, 2019, vol. 5, no. 1. Art. no. 30. https://doi.org/10.1038/s41572-019-0079-y
- Leoni V., Caccia C. The Impairment of Cholesterol Metabolism in Huntington Disease. Biochim. Biophys. Acta, 2015, vol. 1851, no. 8, pp. 1095–1105. https://doi.org/10.1016/j.bbalip.2014.12.018
- Grajales-Reyes G.E., Báez-Pagán C.A., Zhu H., Grajales-Reyes J.G., Delgado-Vélez M., García-Beltrán W.F., Luciano C.A., Quesada O., Ramírez R., Gómez C.M., Lasalde-Dominicci J.A. Transgenic Mouse Model Reveals an Unsuspected Role of the Acetylcholine Receptor in Statin-Induced Neuromuscular Adverse Drug Reactions. Pharmacogenomics J., 2013, vol. 13, no. 4, pp. 362–368. https://doi.org/10.1038/tpj.2012.21
- Crespi B., Alcock J. Conflicts Over Calcium and the Treatment of COVID-19. Evol. Med. Public Health, 2021, vol. 9, no. 1, pp. 149–156. https://doi.org/10.1093/emph/eoaa046
- Ramadan J.W., Steiner S.R., O’Neill C.M., Nunemaker C.S. The Central Role of Calcium in the Effects of Cytokines on Beta-Cell Function: Implications for Type 1 and Type 2 Diabetes. Cell Calcium, 2011, vol. 50, no. 6, pp. 481–490. https://doi.org/10.1016/j.ceca.2011.08.005
- Luciani D.S., Gwiazda K.S., Yang T.L., Kalynyak T.B., Bychkivska Y., Frey M.H., Jeffrey K.D., Sampaio A.V., Underhill T.M., Johnson J.D. Roles of IP3R and RyR Ca2+ Channels in Endoplasmic Reticulum Stress and β-Cell Death. Diabetes, 2009, vol. 58, no. 2, pp. 422–432. https://doi.org/10.2337/db07-1762
- Berchtold M.W., Brinkmeier H., Müntener M. Calcium Ion in Skeletal Muscle: Its Crucial Role for Muscle Function, Plasticity, and Disease. Physiol. Rev., 2000, vol. 80, no. 3, pp. 1215–1265. https://doi.org/10.1152/physrev.2000.80.3.1215
- Guo T., Fan Y., Chen M., Wu X., Zhang L., He T., Wang H., Wan J., Wang X., Lu Z. Cardiovascular Implications of Fatal Outcomes of Patients with Coronavirus Disease 2019 (COVID-19). JAMA Cardiol., 2020, vol. 5, no. 7, pp. 811–818. https://doi.org/10.1001/jamacardio.2020.1017
- Arentz M., Yim E., Klaff L., Lokhandwala S., Riedo F.X., Chong M., Lee M. Characteristics and Outcomes of 21 Critically Ill Patients with COVID-19 in Washington State. JAMA, 2020, vol. 323, no. 16, pp. 1612–1614. https://doi.org/10.1001/jama.2020.4326
- Dani M., Dirksen A., Taraborrelli P., Torocastro M., Panagopoulos D., Sutton R., Lim P.B. Autonomic Dysfunction in ‘Long COVID’: Rationale, Physiology and Management Strategies. Clin. Med. (Lond.), 2021, vol. 21, no. 1, pp. e63–e67. https://doi.org/10.7861/clinmed.2020-0896
- Jung F., Krüger-Genge A., Franke R.P., Hufert F., Küpper J.H. COVID-19 and the Endothelium. Clin. Hemorheol. Microcirc., 2020, vol. 75, no. 1, pp. 7–11. https://doi.org/10.3233/ch-209007
- Jardine D.L., Wieling W., Brignole M., Lenders J.W.M., Sutton R., Stewart J. The Pathophysiology of the Vasovagal Response. Heart Rhythm, 2018, vol. 15, no. 6, pp. 921–929. https://doi.org/10.1016/j.hrthm.2017.12.013
- Chung S.A., Yuan H., Chung F. A Systemic Review of Obstructive Sleep Apnea and Its Implications for Anesthesiologists. Anesth. Analg., 2008, vol. 107, no. 5, pp. 1543–1563. https://doi.org/10.1213/ane.0b013e318187c83a
- Monahan K.D. Effect of Aging on Baroreflex Function in Humans. Am. J. Physiol. Regul. Integr. Comp. Physiol., 2007, vol. 293, no. 1, pp. R3–R12. https://doi.org/10.1152/ajpregu.00031.2007
|
Make a Submission
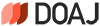
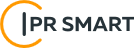
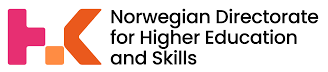
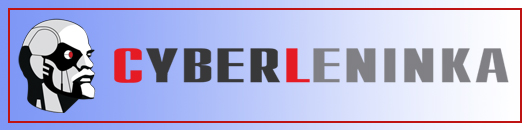
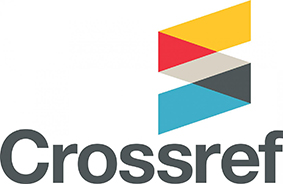
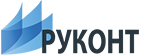
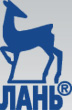
Vestnik of NArFU.
Series "Humanitarian and Social Sciences"
Forest Journal
Arctic and North
|